NARSIMHAN SOFT MATTER RESEARCH GROUP
Purdue Chemical Engineering
(765) 494-4281 (OFFICE)

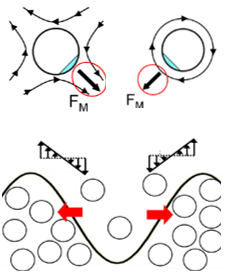
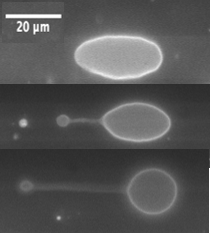



RESEARCH
Soft matter such as biological membranes, tissues, body fluids, colloidal dispersions, and gels have properties that fall between those of liquids and solids. These materials are composed of polymers and/or particulates (liquid or solid) dispersed in a continuous phase. Biological processes and technological applications often use flow and external forces to alter the organization of soft matter at the microscale, which manifests as significant changes in the material’s mechanical behavior. Our group is interested in developing theory and simulations to describe the micro to macroscale behavior of soft matter such as polymeric and biological fluids. We will complement these studies with experiments. We also focus on fundamental transport modeling of relevant industrial processes such as lyophilization that involve simultaneous heat and mass transfer through complex geometries.
RHEOLOGY OF COMPLEX INTERFACES
Many interfaces have mechanical properties that cannot be described by a constant surface tension. Examples include biomembranes, biofilms, and pickering emulsions. In these examples, the elasticity of the films give rise to unique non-equilibrium dynamics under external forcing. For example, red blood cells and vesicles deform/breakup quite differently than droplets under flow due to the presence of a membrane that contains a lipid bilayer and/or surfactant monolayer. Similarly, the elasticity of proteins and polymers in biofilms alter the film’s mechanical stability and growth behavior.  A few questions we would like to address are: can we develop models to describe the dynamics of dispersions with complex interfaces, such as vesicles, red blood cells, and solid-stabilized droplets? How does one model and interpret mechanical measurements of multicomponent interfaces, such as lipid rafts, phase-separated surfactants, etc? Such theories will enable the design of dispersions for consumer products, oil recovery, and nanotechnology, as well as providing insights into biofilms and biological membranes.
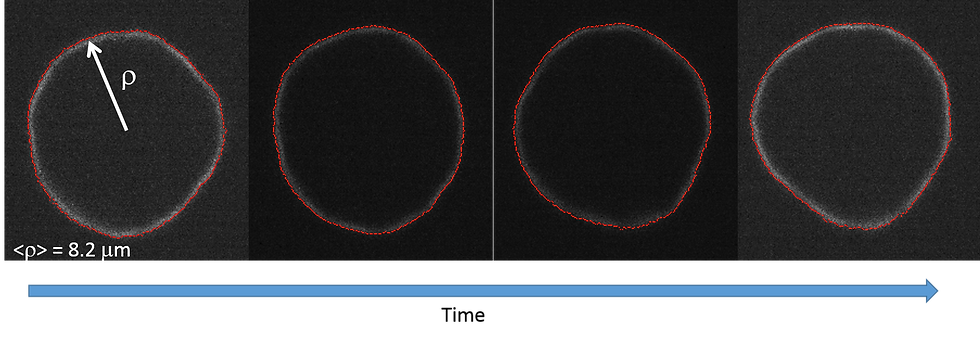


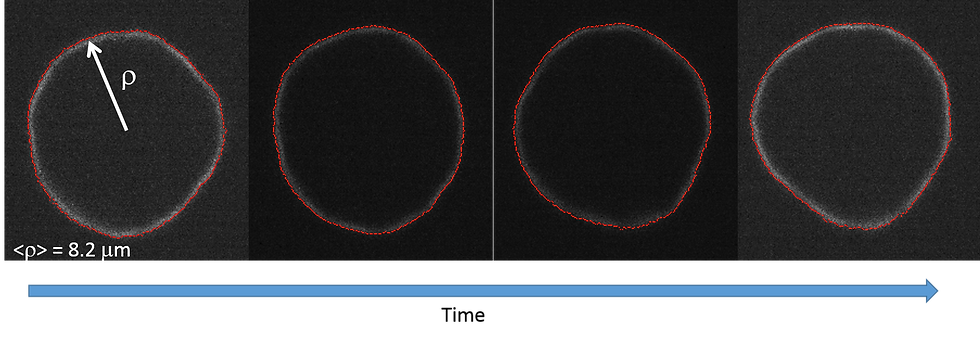
DYNAMICS OF SUSPENSIONS
Suspensions are mixtures of immiscible particulates in a continuous medium such as water. Everyday examples include blood, paint, and aerosols. In many biological and industrial applications, suspensions are multicomponent and it is advantageous to develop efficient techniques to separate each species. We will perform a combination of theory, simulations, and microfluidic experiments to describe the physics of particulate transport in complex (viscoelastic) fluids and concentrated suspensions, particularly when the geometry of the flow channel is complex and transient effects are important. Such information would be valuable in developing separation methods based on particle shape and size, as well describing the motion of blood, cells, and platelets in the micro-circulation.


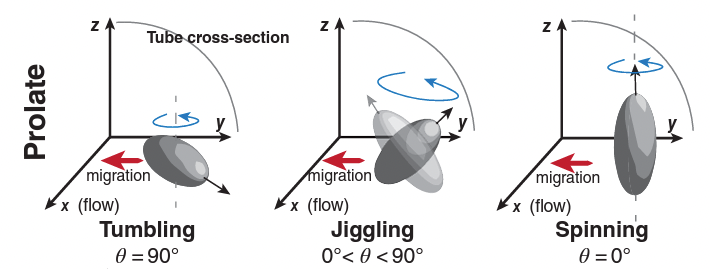

HEAT AND MASS TRANSFER IN PHARMACEUTICAL SYSTEMS
Lyophilization – aka freeze drying – is a common process in pharmaceutical manufacturing where water is removed from a drug formulation to create a shelf-stable solid. This process confers long-term stability to the product, allowing the drug to be stored at room temperature for months on end with little to no degradation. This process involves convective as well as conductive heat and mass transfer in the system. It is important to model such systems and come up with meaningful relations between bulk parameters like the heat transfer coefficient and the microscopic properties like morphology, size, and nature of the material.
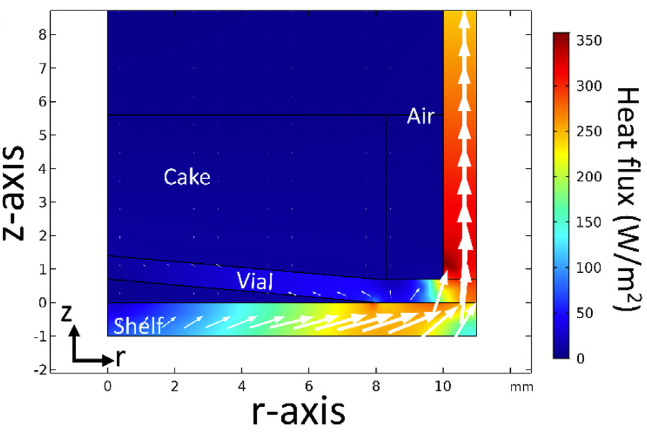
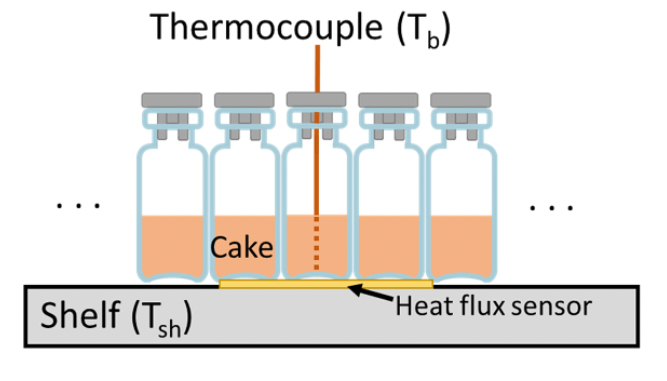
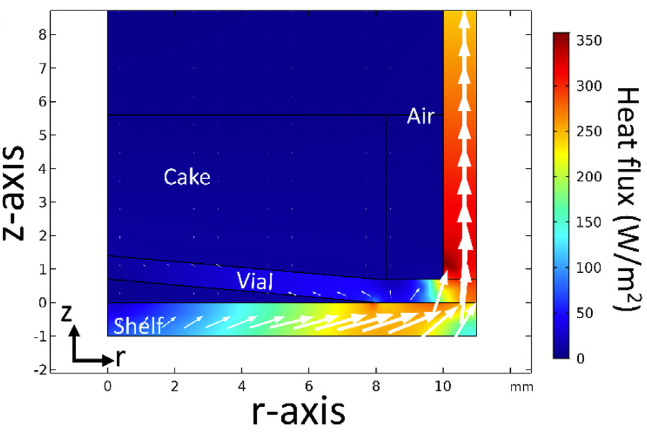
STOCHASTIC PROCESSES
Continuous random walks—i.e.,diffusion with drift—are omni present in a wide range of chemical fields, such as sampling polymer conformations, studying chemical reaction pathways and molecular/Brownian dynamics simulations. In many practical situations, one seeks to quantify random walks whose paths are constrained to stay in a given region, hit a region before another one, or end in a given region. Such ideas allow one to quantify rare events in a chemical process or conversely quantify most probable configurations and reaction pathways.
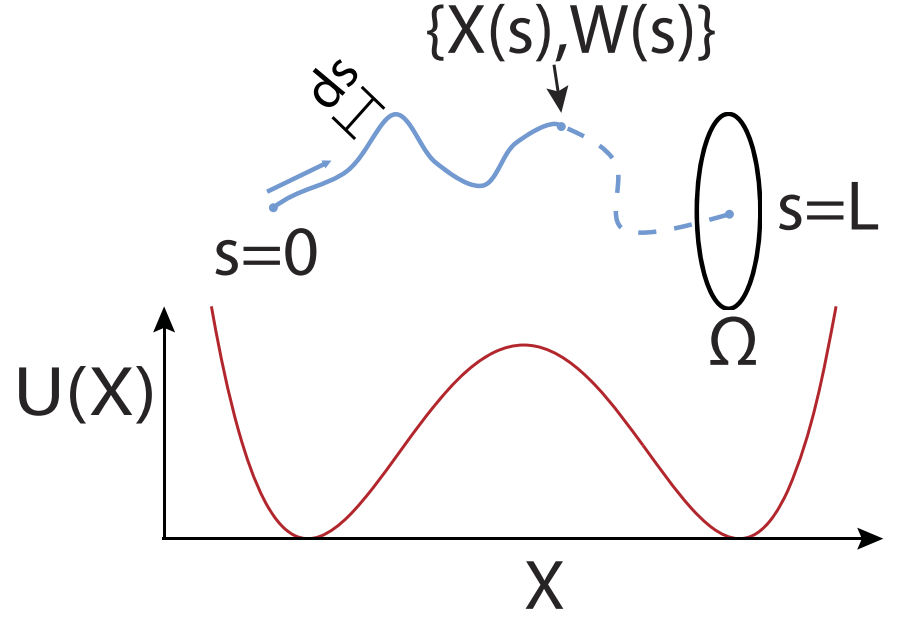
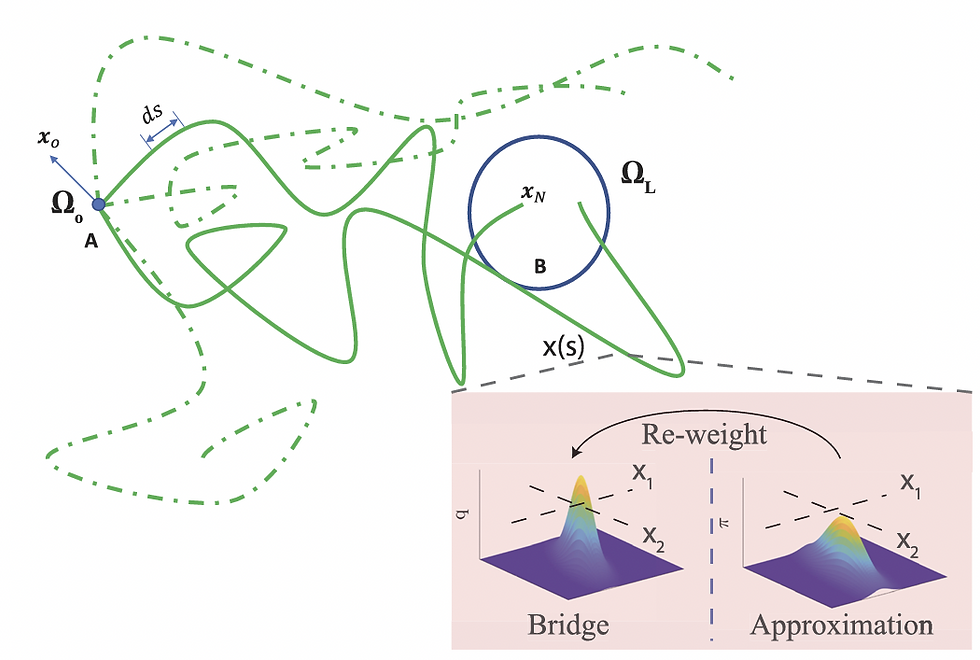
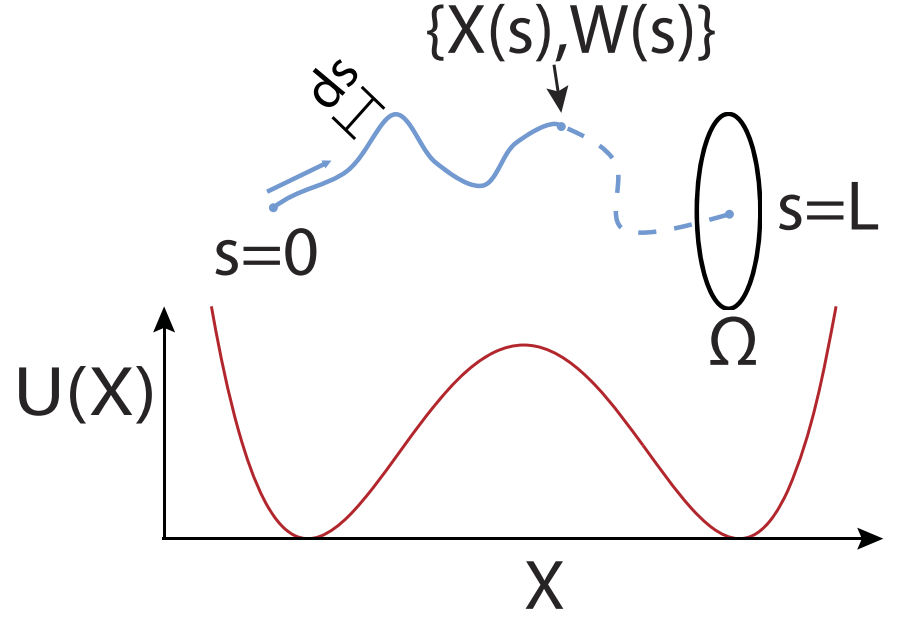